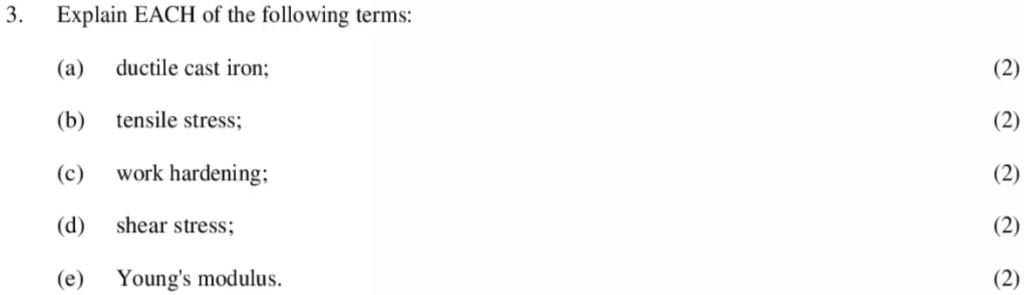
Explain EACH of the following terms:
(a) ductile cast iron;(2)
(b) tensile stress;(2)
(c) work hardening;(2)
(d) shear stress;(2)
(e) Young’s modulus.(2)
Ductile Cast Iron
Ductile cast iron, also known as nodular cast iron or spheroidal graphite iron, is a type of cast iron that boasts significantly improved properties compared to traditional gray cast iron.
2. Ductile Iron – Metal Casting – Reliance Foundry
Key Characteristics:
- Graphite Structure: Unlike gray cast iron, which has flaky graphite inclusions, ductile cast iron contains graphite in spherical nodules. This structure gives it superior strength and ductility. 1. Characterization of the roundness degree of graphite nodules in ductile iron: A new discrete measure independent to resolution – SciELO México www.scielo.org.mx2. The Differences Between Cast Iron and Ductile Iron – McWane Poles www.mcwanepoles.com
- Strength and Ductility: Due to its microstructure, ductile cast iron exhibits high tensile strength, impact resistance, and elongation. It can be bent, twisted, or deformed without fracturing. 1. Properties of Ductile Iron | Saint-Gobain PAM UK www.saint-gobain-pam.co.uk2. Ductile Iron vs Cast Iron: What’s the Difference? www.urick.net
- Versatility: It can be produced with a wide range of properties by controlling its microstructure, making it suitable for various applications. 1. (PDF) DUCTILE IRON MICROSTRUCTURE DESIGN USING HEAT TREATMENT PROCESSES – ResearchGate www.researchgate.net
Benefits of Ductile Cast Iron:
- High strength-to-weight ratio 1. Ductile Iron Casting www.urick.net
- Excellent castability
- Good machinability 1. Machining Advantages of Ductile Iron | ASTM A536 – Penticton Foundry www.pentictonfoundry.com
- Resistance to wear and corrosion 1. Traditional Cast Iron vs. Ductile Iron: Which is More Durable? – Crescent Foundry www.crescentfoundry.com
- Fatigue resistance 1. Ductile iron – Wikipedia en.wikipedia.org
- Impact toughness
Common Applications:
- Automotive components (brake drums, crankshafts, cylinder blocks) 1. Ductile iron – Wikipedia en.wikipedia.org
- Agricultural machinery 1. Why Ductile Iron for Agricultural Castings? www.urick.net
- Construction equipment
- Pipe fittings and valves 1. Ductile vs. Cast Iron Valves: What’s the Difference? – Commercial Industrial Supply www.commercial-industrial-supply.com
- Pump components
In essence, ductile cast iron is a versatile material that offers a combination of strength, ductility, and castability, making it a preferred choice for many engineering applications.
Ductile Cast Iron in Marine Applications
Ductile cast iron’s superior strength, toughness, and castability make it a valuable material in the marine industry. It’s employed in various components due to its ability to withstand harsh marine environments.
Common Applications:
- Marine Engine Components:
- Cylinder blocks and heads: Its strength and heat resistance make it suitable for high-pressure and temperature conditions.
- Crankshafts: Its combination of strength and toughness is ideal for withstanding torsional loads and vibrations.
- Gearboxes: Ductile iron gears provide durability and resistance to wear.
- Hull and Deck Components:
- Anchor and mooring equipment: Its strength and toughness are crucial for handling heavy loads and impacts.
- Pipe fittings and valves: Its corrosion resistance and pressure handling capabilities make it suitable for marine piping systems.
- Propeller hubs and shafts: Its ability to withstand shock loads and vibrations is essential for these components.
- Offshore Structures:
- Subsea equipment: Its strength and corrosion resistance make it suitable for underwater components.
- Platform components: Ductile iron can be used in various structural elements due to its weight-to-strength ratio.
In essence, ductile cast iron’s combination of properties makes it a versatile material for marine applications, contributing to the reliability and durability of marine vessels and equipment.
Tensile Stress
Tensile stress is the force per unit area that tends to stretch a material. Imagine pulling on a rubber band; the force you apply is creating tensile stress in the rubber band.
1. Tensile Stress: Definition, Formula, Unit, Calculation, and Examples | Xometry
2. Stress, Strain, and Elastic Modulus – University Physics Volume 1 – BCcampus Pressbooks
Key Points:
- Force: The pulling force applied to the material. 1. Tensile Stress – Definition, Formula, Example, Unit, Meaning – BYJU’S byjus.com
- Area: The cross-sectional area of the material perpendicular to the force. 1. How does the cross-sectional area of an object affect stress? – TutorChase www.tutorchase.com
- Stress: The ratio of the force to the area. 1. Tensile Stress: Definition, Formula, Unit, Calculation, and Examples | Xometry www.xometry.com
Formula:
Tensile stress (σ) = Force (F) / Area (A)
Units:
Work Hardening
Work hardening, also known as strain hardening or cold working, is the process by which a metal’s strength increases as it is plastically deformed. This means that the metal becomes harder and stronger as it is shaped or formed.
How it works:
- Plastic deformation: When a metal is subjected to forces that exceed its elastic limit, it deforms permanently. 1. What Is Elastic Limit? Definition, Importance, How It Works, and Examples – Xometry www.xometry.com
- Dislocation movement: This deformation causes dislocations (defects in the crystal structure) to move and interact. 1. Dislocation – Wikipedia en.wikipedia.org
- Dislocation entanglement: As deformation continues, dislocations become tangled, making it more difficult for them to move.
- Increased strength: This increased resistance to dislocation movement results in a higher yield strength and increased hardness.
Examples of work hardening processes:
- Rolling 1. Work hardening | Heat Treatment, Strengthening & Tempering – Britannica www.britannica.com
- Drawing
- Forging
- Bending 1. Work hardening – Wikipedia en.wikipedia.org
It’s important to note that work hardening often reduces ductility, meaning the material becomes less able to deform plastically before fracture.
1. Work Hardening & Strain Hardening – A Machinist’s Guide – Tormach
Work Hardened Components in Marine Engineering
While marine engineering often involves components subjected to significant stress, work hardening isn’t as prevalent as in other industries due to the challenges associated with large-scale cold working of marine components. However, there are specific instances where work hardening is utilized:
Potential Components:
- Wire and Cable: These components are often work hardened to increase their strength and resistance to fatigue. This is particularly important for mooring lines, anchor chains, and lifting equipment.
- Fasteners: Bolts, nuts, and other fasteners can be work hardened to improve their resistance to loosening under vibration and cyclic loading.
- Shafts and Axles: In some cases, small-diameter shafts and axles might undergo cold working to enhance their surface hardness and wear resistance.
- Propeller Blades: Although not a typical application, high-performance propellers might utilize work hardening techniques on specific sections to improve cavitation resistance.
Challenges and Alternatives:
- Component Size: The large size of many marine components often precludes effective work hardening.
- Complex Shapes: Many marine components have intricate shapes that make cold working impractical.
As a result, alternative strengthening methods, such as heat treatment, alloying, and surface hardening, are more commonly used in marine engineering.
Shear Stress
Shear stress is the component of stress coplanar with a material cross-section. It arises from a force acting parallel to the surface, causing the material to deform by sliding or shearing.
2. Shear Stress: Definition, How it Works, Example, and Advantages – Xometry
Key points:
- Force: Acts parallel to the material’s surface. 1. Shear stress – Wikipedia en.wikipedia.org
- Area: The area of the surface being acted upon. 1. Shearing Stress – Definition, Examples, Units, Formula, Meaning – BYJU’S byjus.com
- Stress: The ratio of the force to the area. 1. Shear stress – Wikipedia en.wikipedia.org
Formula:
Shear stress (τ) = Force (F) / Area (A)
Units:
- Force: Newtons (N)
- Area: square meters (m²) 1. Shearing Stress – Definition, Examples, Units, Formula, Meaning – BYJU’S byjus.com
- Shear stress: Pascals (Pa) or N/m² 1. Shear stress – Wikipedia en.wikipedia.org
Components Susceptible to Shear Stress in Marine Engineering
Shear stress is a common force encountered in marine engineering due to the dynamic nature of the marine environment and the complex loading conditions experienced by marine structures and equipment. Here are some key components susceptible to shear stress:
Hull Structure
- Transverse and longitudinal bulkheads: These structures experience shear forces due to wave actions, cargo loading, and other external forces.
- Deck and bottom plating: Shear stresses occur due to bending moments and torsional loads.
- Frames and stiffeners: These structural members are subjected to shear forces in conjunction with bending and compressive stresses.
Propulsion Systems
- Shafts: Torque transmission induces shear stresses within the shaft material.
- Propellers: The blades experience shear forces due to water resistance and thrust generation.
- Couplings: These components transmit torque, resulting in shear stresses.
Other Components
- Piping systems: Fluid flow and pressure fluctuations can induce shear stresses in pipes and fittings.
- Marine equipment: Components such as winches, cranes, and hydraulic systems are subject to shear stresses during operation.
Environmental Factors
- Wave and current forces: These forces exert shear stresses on the hull and other submerged structures.
- Ice loading: In regions with ice, ship hulls experience shear forces due to ice impact.
Understanding shear stress distribution is crucial for ensuring the structural integrity and safety of marine vessels. Engineers use advanced analysis techniques, such as finite element analysis, to evaluate shear stress levels and optimize designs.
Young’s Modulus
Young’s modulus is a measure of the stiffness of a solid material. It defines the relationship between stress (force per unit area) and strain (deformation) in the elastic region of a material. In simpler terms, it tells us how much a material will stretch or compress under a given amount of force.
1. Young’s modulus – Wikipedia
3. 12.3 Stress, Strain, and Elastic Modulus | University Physics Volume 1
4. Physics A level revision resource: Introduction to Young’s Modulus – University of Birmingham
Key points:
- Stiffness: A higher Young’s modulus indicates a stiffer material. 1. Shear Modulus vs Young’s Modulus: Which One to Use? – Xometry www.xometry.com
- Elastic region: The material returns to its original shape after the load is removed. 1. 12.4 Elasticity and Plasticity | University Physics Volume 1 – Courses.lumenlearning.com. courses.lumenlearning.com
- Calculation: Young’s modulus (E) = stress (σ) / strain (ε) 1. 12.3 Stress, Strain, and Elastic Modulus – University Physics Volume 1 | OpenStax openstax.org
Young’s Modulus in Marine Engineering
Young’s modulus is a critical parameter in marine engineering, as it directly relates to the stiffness and deformation of materials under load. Its applications include:
Structural Design
- Hull Stiffness: Determining the amount of deflection a ship’s hull will experience under wave loads.
- Beam and Column Design: Calculating the deflection of structural members like beams and columns under various loading conditions. 1. Young’s modulus – Wikipedia en.wikipedia.org
- Material Selection: Choosing materials with appropriate Young’s modulus for different components based on required stiffness and weight considerations.
Equipment Design
- Shaft and Propeller Design: Calculating torsional stiffness and deflection of shafts and propellers.
- Subsea Equipment: Designing components that can withstand high pressures without significant deformation.
Material Selection
- Selecting Materials: Choosing materials with suitable Young’s modulus for specific applications, such as high-strength, low-weight components.
- Composite Materials: Characterizing the elastic behavior of composite materials used in marine structures.
Finite Element Analysis (FEA)
- Structural Analysis: Incorporating Young’s modulus into FEA models to predict stress and strain distributions.
- Optimization: Using FEA to optimize material selection and structural design based on stiffness requirements.
By understanding and utilizing Young’s modulus, marine engineers can design safer, more efficient, and reliable marine structures and equipment.