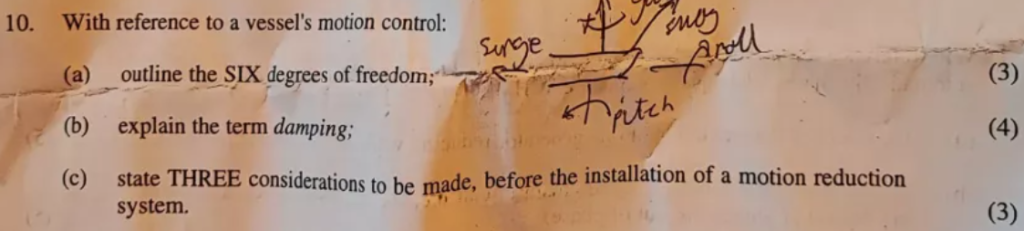
- With reference to a vessel’s motion control:
(a) outline the SIX degrees of freedom;
(b) explain the term damping;(4)
(c) state THREE considerations to be made, before the installation of a motion reduction system.(3)
SIX degrees of freedom
In the context of a vessel’s motion control, the six degrees of freedom (6DOF) refer to the six fundamental types of movement that a vessel can experience in three-dimensional space. These movements are categorized into three translational movements and three rotational movements. Understanding these motions is crucial for controlling a ship’s stability, maneuverability, and response to external forces such as waves, wind, and currents.
The Six Degrees of Freedom:
1. Surge (Translational Movement)
- Description: Surge is the forward and backward motion of the vessel along its longitudinal axis (the axis that runs from the bow to the stern).
- Effect: Surge refers to the vessel’s acceleration or deceleration in the direction of its forward motion (along the x-axis). For example, when a vessel moves forward through the water, it is experiencing surge.
- Control: Surge is controlled by adjusting the vessel’s propulsion system (e.g., engine power and thrust).
2. Sway (Translational Movement)
- Description: Sway is the side-to-side movement of the vessel along its lateral axis (the axis that runs from port to starboard).
- Effect: Sway occurs when external forces, such as wind, waves, or currents, push the vessel sideways (along the y-axis). This motion is common when a vessel is docking or in a cross-current.
- Control: Sway can be controlled using bow thrusters, stern thrusters, or rudder adjustments.
3. Heave (Translational Movement)
- Description: Heave is the up-and-down vertical movement of the vessel along its vertical axis (the axis running from the keel to the top of the vessel).
- Effect: Heave is caused by the vessel moving in response to vertical forces from waves or sea conditions. It is a common motion when a vessel is navigating rough seas.
- Control: Heave cannot be directly controlled by the vessel, but the design of the hull and stabilization systems (such as active stabilizers) can minimize its effects.
4. Roll (Rotational Movement)
- Description: Roll is the rotational movement of the vessel around its longitudinal axis (bow to stern), causing the vessel to tilt from side to side.
- Effect: Roll occurs when the vessel rocks back and forth on its side (along the x-axis), typically due to wave action, causing the vessel to list toward port or starboard.
- Control: Roll is managed using stabilizers (e.g., fin stabilizers, gyroscopic stabilizers) or by adjusting the vessel’s speed and heading relative to the waves.
5. Pitch (Rotational Movement)
- Description: Pitch is the rotational movement of the vessel around its lateral axis (port to starboard), causing the vessel’s bow and stern to move up and down.
- Effect: Pitch occurs when the bow rises and falls (along the y-axis) due to waves, especially when a vessel is moving head-on into rough seas.
- Control: Pitch can be influenced by adjusting the vessel’s speed and heading, but like roll, it is also managed using stabilizers.
6. Yaw (Rotational Movement)
- Description: Yaw is the rotational movement of the vessel around its vertical axis (keel to the top of the vessel), causing the vessel to turn to port or starboard.
- Effect: Yaw occurs when the vessel’s heading changes direction (along the z-axis). It is the most controlled motion in the context of steering, as it determines the vessel’s course.
- Control: Yaw is controlled using the vessel’s rudder, azimuth thrusters, or other steering mechanisms.
Summary of the Six Degrees of Freedom:
- Translational Motions (Linear Movements):
- Surge: Forward and backward motion along the longitudinal axis (bow-stern).
- Sway: Side-to-side motion along the lateral axis (port-starboard).
- Heave: Up-and-down motion along the vertical axis (keel to top).
- Rotational Motions (Angular Movements): 4. Roll: Tilting side-to-side around the longitudinal axis (port-starboard rocking). 5. Pitch: Up-and-down rotation around the lateral axis (bow-stern rocking). 6. Yaw: Turning left or right around the vertical axis (changing heading to port or starboard).
Importance in Vessel Motion Control:
Understanding the six degrees of freedom is crucial for designing and operating vessels in a stable and efficient manner. Shipbuilders use this knowledge to design hulls and stabilizing systems to minimize undesirable motions, while navigators and ship operators use motion control strategies to maintain course, stability, and comfort for passengers and cargo during a voyage. Stabilizers, rudders, and thrusters are key components in managing these degrees of freedom, especially in challenging sea conditions.
Damping
In the context of a vessel’s motion control, damping refers to the process of reducing or mitigating the amplitude of a vessel’s oscillatory or vibratory motion caused by external forces such as waves, wind, and currents. Damping is essential in minimizing the undesirable effects of the vessel’s movements, particularly in the six degrees of freedom (surge, sway, heave, roll, pitch, and yaw), which can affect the vessel’s stability, safety, and comfort.
Key Concepts of Damping:
- Oscillatory Motion in Vessels:
- Vessels are constantly exposed to external forces like waves, which induce oscillations or repetitive movements such as rolling, pitching, and yawing.
- These oscillations can persist and even increase in amplitude if not controlled, leading to discomfort for crew and passengers, potential damage to cargo, and increased stresses on the vessel’s structure.
- Damping Effect:
- Damping is the mechanism by which energy from these oscillatory motions is dissipated or absorbed, resulting in a gradual reduction of the amplitude of the motion over time.
- Damping does not prevent motion but reduces its intensity and duration, helping to stabilize the vessel more quickly.
Types of Damping in Vessel Motion Control:
1. Hydrodynamic Damping
- Description: Hydrodynamic damping is caused by the interaction between the vessel’s hull and the surrounding water. As the vessel moves through or oscillates in the water, the water exerts forces on the hull that resist the motion, naturally reducing the amplitude of the oscillations.
- Example: When a vessel rolls, the water around the hull generates resistance (drag), which helps slow down and reduce the rolling motion.
- Effect: Hydrodynamic damping is an inherent property of the hull design and shape. Hulls with larger wetted surface areas generally experience more damping due to greater water resistance.
2. Viscous Damping
- Description: Viscous damping is related to the frictional resistance between the vessel and the surrounding water. The viscosity (thickness) of the water generates drag forces that oppose the vessel’s movement and help absorb energy from oscillations.
- Example: As a vessel moves through the water, the friction between the water and the hull resists the motion, slowing down rolling or pitching.
- Effect: Viscous damping is dependent on the water conditions and the surface area of the hull in contact with the water.
3. Structural Damping
- Description: Structural damping refers to the dissipation of energy within the vessel’s structure (hull, superstructure, etc.) as it flexes or deforms in response to motion. Internal friction between different structural components absorbs energy, helping to reduce the intensity of the motion.
- Example: When the ship rolls or pitches, some of the energy is absorbed by the flexing of the hull or other structural elements, reducing the overall motion.
- Effect: Structural damping is generally small but contributes to the overall damping effect, especially in larger or more flexible vessels.
4. Active Damping Systems (Stabilizers)
- Description: Active damping systems are technologies designed to actively counteract the effects of unwanted vessel motions, particularly roll and pitch. These systems include devices like fin stabilizers, gyroscopic stabilizers, and anti-roll tanks.
- Example:
- Fin Stabilizers: These are wing-like fins mounted on the vessel’s hull below the waterline. They move automatically to counteract the rolling motion by generating lift in the opposite direction of the roll.
- Gyroscopic Stabilizers: These use the principles of gyroscopic motion to generate forces that resist rolling.
- Anti-Roll Tanks: These are water-filled tanks that move water from side to side to counterbalance the roll.
- Effect: Active damping systems significantly reduce the rolling and pitching motions, enhancing vessel stability and comfort.
5. Natural Damping
- Description: Natural damping refers to the inherent ability of the vessel to dampen its own motion without external or mechanical assistance. This can depend on factors like hull design, weight distribution, and the vessel’s operational speed.
- Example: A well-designed hull with a low center of gravity and good stability characteristics will naturally dampen roll and pitch more effectively.
- Effect: The natural damping ability of a vessel is a key consideration in ship design, as it helps reduce the need for extensive mechanical damping systems.
Importance of Damping in Vessel Motion Control:
- Improving Stability: Damping plays a critical role in stabilizing the vessel by reducing unwanted motions, especially in rough seas. This helps maintain the vessel’s equilibrium and prevents extreme angles of roll or pitch.
- Enhancing Safety: Excessive motion can endanger the vessel’s crew, passengers, and cargo. Proper damping reduces the likelihood of accidents, such as cargo shifts or crew falls, and decreases the risk of structural damage due to excessive stress.
- Increasing Comfort: For passenger vessels, cruise ships, and yachts, damping is essential to minimize uncomfortable rolling or pitching, making the voyage smoother and more enjoyable.
- Reducing Fatigue on the Vessel’s Structure: Continuous oscillatory motions can cause fatigue and wear on the vessel’s structure over time. Damping helps limit these movements, thereby reducing the stresses on the hull and components, prolonging the lifespan of the ship.
- Energy Efficiency: Damping helps reduce the need for excessive corrective maneuvers by the crew or automatic systems. In the case of active damping systems, this can result in more efficient energy usage by reducing drag and unnecessary fuel consumption.
Conclusion:
Damping in vessel motion control is the process of reducing oscillatory motions like roll, pitch, and yaw, which are caused by external forces such as waves and wind. Damping occurs through natural hydrodynamic resistance, structural flexibility, and the use of active stabilizing technologies. It is essential for improving vessel stability, safety, and comfort, as well as minimizing the wear and tear on the vessel’s structure and components. Proper damping allows vessels to operate more efficiently and safely in a wide range of sea conditions.
Considerations to be made, before the installation of a motion reduction system.
Before the installation of a motion reduction system (such as fin stabilizers, gyroscopic stabilizers, or anti-roll tanks) on a vessel, several important considerations must be taken into account to ensure the system’s effectiveness, compatibility, and cost-efficiency. These considerations include technical, operational, and financial factors, as well as the specific needs of the vessel based on its size, design, and operational profile.
Key Considerations Before Installing a Motion Reduction System:
1. Vessel Size and Type
- Consideration: The size and type of vessel are critical factors in selecting the appropriate motion reduction system. Larger vessels like cruise ships, cargo ships, or tankers will require different systems than smaller vessels like yachts, fishing boats, or patrol vessels.
- Impact: Larger vessels may benefit from systems like fin stabilizers or anti-roll tanks, which can handle the greater forces involved in large-scale roll and pitch. Smaller vessels may require lighter, less intrusive systems such as gyroscopic stabilizers.
- Example: A large container ship may opt for passive anti-roll tanks, whereas a luxury yacht might install gyroscopic stabilizers for minimal noise and space usage.
2. Operational Profile and Sea Conditions
- Consideration: The operational profile of the vessel, including the routes it takes, the typical sea conditions it encounters, and its average speed, is essential when determining the most appropriate motion reduction system.
- Impact: A vessel operating in rough seas will require a more robust motion reduction system, whereas vessels operating in calmer waters may only need a lighter system. Additionally, vessels traveling at high speeds may benefit from active fin stabilizers, while slower vessels may prefer passive or gyroscopic systems.
- Example: A vessel crossing rough seas regularly would need a more powerful system to deal with extreme pitching and rolling, while a coastal ferry operating in calmer waters may require a simpler system.
3. Hull Design and Configuration
- Consideration: The shape and design of the vessel’s hull are important factors in determining the effectiveness and compatibility of a motion reduction system. The system must be compatible with the hull to ensure proper installation and operation.
- Impact: Some hull shapes are better suited for certain types of systems. For example, long, narrow hulls may benefit more from active fin stabilizers, while vessels with large, flat bottoms might require gyroscopic stabilizers.
- Example: Fin stabilizers require specific hull designs that allow the fins to be mounted without compromising the hull’s integrity or the hydrodynamics of the vessel.
4. Space and Weight Considerations
- Consideration: The available space for installing the motion reduction system is a crucial factor, especially for smaller vessels or retrofitting projects. The weight of the system is also important, as it can affect the vessel’s balance and stability.
- Impact: Systems like gyroscopic stabilizers can be heavy and take up valuable space below deck. On the other hand, fin stabilizers are mounted externally but may require additional space inside for actuators and control mechanisms.
- Example: If space is limited in a small vessel, a gyroscopic stabilizer may need to be placed in a location that does not interfere with other equipment, or alternative systems like interceptors may be considered.
5. Energy Requirements
- Consideration: Some motion reduction systems, such as active fin stabilizers and gyroscopic stabilizers, require significant amounts of energy to operate, which can affect the vessel’s overall energy consumption.
- Impact: Energy-intensive systems will increase the vessel’s fuel consumption, potentially reducing its overall efficiency. Additionally, the power supply onboard must be sufficient to meet the demands of the stabilizing system.
- Example: For a vessel with limited generator capacity, installing a high-energy-consuming system may require upgrading the electrical system, which can be costly and time-consuming.
6. Maintenance and Reliability
- Consideration: The long-term maintenance requirements and reliability of the motion reduction system should be factored in when making a decision. Some systems require more frequent maintenance than others, which can increase operational costs.
- Impact: Systems with moving parts, such as fin stabilizers, may require more frequent maintenance, inspections, and repairs than passive systems like anti-roll tanks. Additionally, the reliability of the system must be considered, as failure during heavy seas could endanger the vessel.
- Example: A vessel with a tight maintenance schedule may prefer a low-maintenance system, such as a passive anti-roll tank, over a high-maintenance active stabilizer.
7. Cost and Budget
- Consideration: The initial cost of purchasing and installing the motion reduction system, along with the long-term operational and maintenance costs, must fit within the vessel’s budget.
- Impact: High-performance systems such as gyroscopic stabilizers or advanced fin stabilizers can be expensive to install and maintain. Vessel owners must weigh the benefits of reduced motion against the overall cost of the system, including any necessary retrofits or modifications to the vessel.
- Example: A commercial vessel operator may prioritize cost-effective solutions with minimal operational costs, while a luxury yacht owner may focus on comfort and performance, despite the higher initial investment.
8. Retrofitting vs. New Build
- Consideration: The decision between installing a motion reduction system on a new build or retrofitting it to an existing vessel affects the complexity of the project. Retrofitting can be more challenging due to space limitations, design constraints, and compatibility issues.
- Impact: Retrofitting may require structural modifications to the vessel, such as reinforcing the hull or reallocating space for the new system, which can increase the cost and time required for installation.
- Example: A new build can be designed with motion control systems in mind from the beginning, allowing for optimal placement and integration. Retrofitting, however, may require compromise due to existing space or design limitations.
9. Impact on Vessel Performance
- Consideration: Some motion reduction systems, such as fin stabilizers, can create additional drag, reducing the vessel’s speed or increasing fuel consumption.
- Impact: Any system that affects the vessel’s hydrodynamics or weight distribution can have an impact on performance. It’s important to ensure that the benefits of motion reduction outweigh any potential performance losses.
- Example: A vessel designed for high-speed operation may need to carefully consider the drag created by fin stabilizers, which could reduce speed and fuel efficiency.
10. Effect on Crew and Passenger Comfort
- Consideration: The primary reason for installing a motion reduction system is to improve comfort for crew and passengers by reducing motion such as roll and pitch. The effectiveness of the system in enhancing comfort should be evaluated based on the vessel’s operational environment and the level of comfort required.
- Impact: Systems like gyroscopic stabilizers are highly effective at reducing roll and improving comfort in calm to moderate seas, while fin stabilizers are more effective at high speeds.
- Example: For a cruise ship or yacht, passenger comfort is a priority, and a highly effective motion reduction system like gyroscopes might be the best choice.
Conclusion:
Before installing a motion reduction system on a vessel, it is essential to consider factors such as the vessel’s size and type, operational profile, space and weight limitations, energy requirements, cost, and maintenance needs. Additionally, the impact of the system on vessel performance and passenger comfort, as well as the feasibility of retrofitting versus new installations, should be carefully evaluated. By considering these factors, vessel owners and operators can choose the most appropriate system to improve stability and comfort while balancing cost, performance, and operational efficiency.