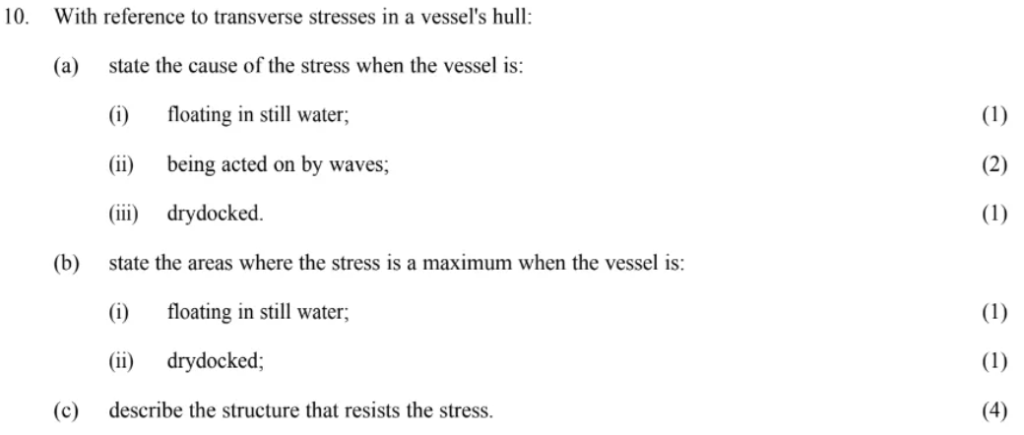
- With reference to transverse stresses in a vessel’s hull:
(a) state the cause of the stress when the vessel is:
(i) floating in still water;(1)
(ii) being acted on by waves;(2)
(iii) drydocked.(1)
(b) state the areas where the stress is a maximum when the vessel is:
(i) floating in still water;(1)
(ii) drydocked;(1)
(c) describe the structure that resists the stress.(4)
What are transverse stresses in a vessel’s hull?
Transverse stresses in a vessel’s hull are forces acting across the width of the ship, perpendicular to its longitudinal axis (the line running from bow to stern). These stresses tend to distort the ship’s shape in a horizontal plane, potentially causing deformation or even structural failure if not adequately addressed.
Main Types of Transverse Stresses:
- Racking Stresses:
- Cause: Primarily caused by the rolling motion of the ship in a seaway. As the vessel rolls, the upper parts of the hull (deck and superstructure) tend to move laterally relative to the lower parts (keel and bottom), creating a twisting or racking effect.
- Effect: This puts stress on the corners of the hull, particularly at the connections between the deck beams and side frames (beam knees), as well as on the shell plating and bulkheads.
- Water Pressure:
- Cause: The hydrostatic pressure exerted by the water on the submerged portion of the hull. This pressure increases with depth and acts perpendicular to the hull surface.
- Effect: Creates compressive stresses that try to “squeeze” the hull inwards. These stresses are highest at the ship’s bottom and decrease towards the waterline.
- Internal Loading:
- Cause: The weight of the cargo, machinery, equipment, and other items within the ship, as well as the weight of the hull structure itself.
- Effect: Creates varying stresses depending on the distribution of the load. Concentrated loads, such as heavy machinery or cargo, can cause localized stresses on the hull structure.
- Docking Stresses:
- Cause: When a ship is in dry dock, the supporting force of the water is removed, and the hull is supported by blocks.
- Effect: This can cause the hull to sag downwards on either side of the keel, putting the deck beams in tension and the bottom structure in compression.
Importance of Considering Transverse Stresses:
- Structural Design: Ship designers must carefully consider transverse stresses when determining the scantlings (dimensions and thickness) of the hull plating, frames, beams, and bulkheads to ensure adequate strength and prevent excessive deformation or failure.
- Stability: Transverse stresses can also affect the ship’s stability, particularly racking stresses that can cause a twisting motion.
- Safety: Excessive transverse stresses can lead to structural damage, leaks, or even catastrophic failures, compromising the safety of the vessel and its crew.
Mitigation Measures:
- Transverse Framing System: The ship’s transverse framing system, consisting of frames, beams, and bulkheads, is designed to resist racking stresses and provide transverse strength.
- Strong Connections: Strong and well-designed connections between the frames, beams, and shell plating are crucial for distributing transverse stresses effectively.
- Proper Load Distribution: Careful planning of cargo and ballast loading helps to minimize uneven loading and reduce localized stresses.
- Dry Dock Support: Adequate support and blocking arrangements in dry dock are essential to prevent excessive sagging and stress on the hull.
In conclusion, understanding and managing transverse stresses is crucial for ensuring the structural integrity, stability, and safety of a vessel. By considering these forces during design and operation, shipbuilders and operators can create and maintain seaworthy vessels capable of withstanding the challenges of the marine environment.
Cause of the stress when the vessel is floating in still water
Even when a vessel is floating in still water, its hull experiences transverse stresses. The primary cause of these stresses is the uneven distribution of weight and buoyancy across the ship’s breadth.
Here’s a breakdown:
- Weight: The ship’s weight isn’t uniformly distributed. Heavier components like engines, machinery, and cargo holds create concentrated loads in specific areas.
- Buoyancy: While the total buoyant force acting upwards equals the ship’s weight, the distribution of buoyancy isn’t always uniform either. This is because the hull’s shape and the underwater volume it displaces can vary across its breadth.
- Stress Concentration: These uneven weight and buoyancy distributions create localized stress concentrations in the hull structure. For example, areas with heavier loads will experience higher stresses, while areas with greater buoyancy might experience less stress or even tension.
Specific Types of Transverse Stresses in Still Water:
- Water Pressure:
- Cause: The hydrostatic pressure exerted by the water on the submerged portion of the hull. This pressure acts perpendicular to the hull surface and increases with depth.
- Effect: Creates compressive stresses that try to “squeeze” the hull inwards. These stresses are highest at the ship’s bottom and decrease towards the waterline.
- Internal Loading:
- Cause: The weight of the cargo, machinery, equipment, and other items within the ship, as well as the weight of the hull structure itself.
- Effect: Creates varying stresses depending on the distribution of the load. Concentrated loads, such as heavy machinery or cargo, can cause localized stresses on the hull structure, potentially leading to bending or deformation.
- Uneven Buoyancy:
- Cause: Variations in the hull shape or the presence of appendages like bilge keels can create uneven buoyancy distribution.
- Effect: This can lead to localized stresses, particularly in areas where the buoyant force is concentrated or where there’s a sudden change in hull shape.
Importance of Considering Transverse Stresses in Still Water:
- Structural Design: Ship designers must account for these stresses when determining the scantlings (dimensions and thickness) of the hull plating, frames, and beams to ensure adequate strength and prevent excessive deformation or failure.
- Stability: While less pronounced than in dynamic conditions, transverse stresses in still water can still affect the ship’s stability.
- Long-term Fatigue: Even in calm water, continuous exposure to these stresses can contribute to fatigue and potential cracking or failure of the hull structure over time.
Mitigation Measures:
- Transverse Framing System: The ship’s transverse framing system, consisting of frames, beams, and bulkheads, is designed to resist these stresses and provide transverse strength.
- Strong Connections: Strong and well-designed connections between the frames, beams, and shell plating are crucial for distributing stresses effectively.
- Proper Load Distribution: Careful planning of cargo and ballast loading helps to minimize uneven loading and reduce localized stresses.
In conclusion, even in still water, a vessel’s hull experiences transverse stresses primarily due to the uneven distribution of weight and buoyancy. Understanding and managing these stresses are crucial for ensuring the structural integrity, stability, and long-term durability of the vessel.
Cause of the stress when the vessel is being acted on by waves
When a vessel is acted upon by waves, the transverse stresses on its hull become more complex and dynamic compared to the relatively static stresses experienced in still water. The primary cause of these stresses is the uneven and fluctuating pressure distribution exerted by the waves on the hull’s surface.
Here’s a breakdown of how waves induce transverse stresses:
- Wave Action: As waves pass along the ship’s length, they create varying pressures on the hull. The crest of a wave exerts higher pressure, while the trough exerts lower pressure. This alternating pressure distribution causes the hull to bend and deform in a transverse direction.
- Rolling and Pitching: The ship’s rolling and pitching motions, induced by the waves, further amplify the transverse stresses. As the vessel rolls, the deck and upper portions of the hull tend to move laterally relative to the lower portions, creating racking stresses that distort the hull’s shape.
- Wave Impact: In rough seas, waves can impact the hull with significant force, creating localized stress concentrations and potentially causing damage to the structure.
- Dynamic Loading: The continuous and varying nature of wave action creates dynamic loading on the hull, leading to fatigue stresses that can accumulate over time and potentially weaken the structure.
Specific Types of Transverse Stresses Induced by Waves:
- Racking Stresses:
- Cause: Primarily caused by the rolling motion of the ship in waves.
- Effect: This puts stress on the corners of the hull, particularly at the connections between the deck beams and side frames, as well as on the shell plating and bulkheads.
- Hydrodynamic Pressure:
- Cause: The varying pressure exerted by the waves on the hull surface, which can be significantly higher than the hydrostatic pressure in still water.
- Effect: Creates fluctuating compressive and tensile stresses on the hull, potentially leading to fatigue and cracking over time.
- Wave Impact Loads:
- Cause: Direct impact of waves on the hull, especially in rough seas or when the ship is slamming into waves.
- Effect: Creates high localized stresses that can cause damage to the hull plating, frames, or other structural members.
Importance of Considering Transverse Stresses in Waves:
- Structural Design: Ship designers must carefully consider these dynamic stresses when determining the scantlings (dimensions and thickness) of the hull and its supporting structure to ensure adequate strength and prevent fatigue failures.
- Seakeeping: The ship’s ability to withstand and operate safely in waves (seakeeping) is directly related to its resistance to transverse stresses.
- Safety: Excessive transverse stresses can lead to structural damage, leaks, or even catastrophic failures, compromising the safety of the vessel and its crew.
Mitigation Measures:
- Transverse Framing System: A robust transverse framing system, including frames, beams, and bulkheads, is designed to resist racking stresses and provide transverse strength.
- Strong Connections: Strong and well-designed connections between the frames, beams, and shell plating are crucial for distributing stresses effectively.
- Hull Form and Design: The ship’s hull form and design can also influence its response to waves and the magnitude of transverse stresses.
- Operational Practices: Avoiding heavy weather or adjusting speed and course can help reduce the impact of waves and the associated stresses.
In conclusion, when a vessel is acted upon by waves, the transverse stresses on its hull become dynamic and more complex due to the uneven and fluctuating pressure distribution. Understanding and managing these stresses are crucial for ensuring the structural integrity, seaworthiness, and safety of the vessel in a seaway.
Cause of the stress when the vessel is drydocked
When a vessel is dry-docked, the transverse stresses on its hull arise primarily from the loss of buoyancy support and the concentrated loads imposed by the dry dock blocks.
Here’s a breakdown of the causes:
- Loss of Buoyancy: When a ship is afloat, the water provides distributed support along the entire length of the hull. In dry dock, this buoyant force is removed, and the ship’s weight is concentrated on the keel blocks and side blocks.
- Concentrated Loads: The keel blocks and side blocks exert concentrated forces on the hull structure, primarily at the points of contact. This creates localized stresses that can be significantly higher than the distributed stresses experienced when the ship is afloat.
- Sagging: The hull tends to sag downwards between the supporting blocks, putting the deck beams in tension and the bottom structure in compression. This sagging effect is more pronounced in vessels with longer distances between the blocks or those with weaker transverse framing.
- Uneven Load Distribution: If the vessel is not perfectly aligned on the blocks or if the blocks are not evenly spaced, it can lead to uneven load distribution and increased stresses in certain areas of the hull.
Specific Types of Transverse Stresses in Dry Dock:
- Docking Stresses:
- Cause: The loss of buoyancy support and the concentrated loads from the blocks.
- Effect: Creates significant bending moments and stresses on the hull structure, particularly in the areas between the supporting blocks.
- Internal Loading:
- Cause: The weight of the cargo, machinery, equipment, and other items within the ship, as well as the weight of the hull structure itself.
- Effect: While internal loading is present even when the ship is afloat, its effect on transverse stresses is more pronounced in dry dock due to the loss of buoyancy support.
Importance of Considering Transverse Stresses in Dry Dock:
- Structural Design: Ship designers must account for these stresses when determining the scantlings (dimensions and thickness) of the hull plating, frames, and beams to ensure adequate strength and prevent excessive deformation or damage during dry-docking.
- Docking Plan: A detailed docking plan is essential to specify the proper placement and spacing of the blocks to minimize stresses and ensure safe docking.
- Monitoring and Adjustments: During the docking process, the ship’s position and stresses should be carefully monitored, and adjustments made as needed to prevent excessive sagging or uneven load distribution.
Mitigation Measures:
- Adequate Support: The dry dock should be equipped with a sufficient number of keel blocks and side blocks to distribute the ship’s weight evenly and prevent excessive sagging.
- Proper Block Placement: The blocks should be carefully positioned based on the ship’s docking plan to minimize stress concentrations and ensure adequate support for the hull structure.
- Strong Transverse Framing: A robust transverse framing system, including frames, beams, and bulkheads, helps to resist the docking stresses and maintain the hull’s shape.
- Monitoring and Adjustments: Continuous monitoring of the ship’s position and stress levels during dry-docking allows for timely adjustments to prevent damage.
In conclusion, when a vessel is dry-docked, the transverse stresses on its hull are primarily caused by the loss of buoyancy support and the concentrated loads from the dry dock blocks. Understanding and managing these stresses are crucial for preventing structural damage and ensuring the safe and successful completion of the dry-docking operation.
Areas where the stress is a maximum when the vessel is floating in still water
Even when a vessel is floating in still water, its hull experiences transverse stresses. These stresses are primarily caused by the uneven distribution of weight and buoyancy across the ship’s breadth.
Areas of Maximum Stress:
- Mid-ship Section:
- This is generally the widest part of the ship and experiences the greatest bending moment due to the weight distribution and buoyancy forces.
- The deck and bottom plating at mid-ship are subjected to the highest longitudinal bending stresses (hogging or sagging), which in turn induce transverse stresses in the frames and beams.
- Corners of the Hull:
- The junctions between the deck, sides, and bottom of the hull (often called “beam knees” or “tank side brackets”) are areas of stress concentration.
- These areas experience high stresses due to the combination of longitudinal bending and the tendency of the hull to deform in a transverse direction.
- Areas with Concentrated Loads:
- Locations with heavy machinery, engines, or cargo can experience higher localized transverse stresses due to the concentrated weight.
- This is particularly true for engine room bulkheads and tank tops under heavy machinery.
- Areas with Abrupt Changes in Hull Shape:
- Sudden changes in hull shape, such as at the turn of the bilge or near the stern, can create stress concentrations and higher transverse stresses.
- Bulkhead Connections:
- The points where transverse bulkheads connect to the hull plating and deck can also experience high stresses, especially if the bulkheads are not adequately stiffened or supported.
Why these areas are critical:
- Structural Failure: If these areas are not designed and constructed with sufficient strength, they can be susceptible to cracking, buckling, or even structural failure, potentially leading to leaks or compromising the vessel’s integrity.
- Fatigue: Continuous exposure to transverse stresses, even in still water, can contribute to fatigue cracking over time, especially in areas of stress concentration.
Mitigating Measures:
- Stronger Scantlings: Using thicker plating, larger frames, and closer frame spacing in these high-stress areas can increase their strength and resistance to deformation.
- Reinforcements: Adding extra stiffeners, brackets, or doublers to strengthen the connections and distribute stresses more evenly.
- Proper Load Distribution: Careful planning of cargo and ballast loading to avoid excessive localized loads.
- Regular Inspections: Conducting regular inspections and maintenance to identify and address any signs of wear, corrosion, or damage in these critical areas.
In conclusion, understanding the areas of maximum transverse stress in a vessel’s hull, even in still water, is crucial for ensuring its structural integrity and safety. By addressing these stress concentrations through proper design, construction, and maintenance, shipbuilders and operators can create and maintain seaworthy vessels capable of withstanding the challenges of the marine environment.
Areas where the stress is a maximum when the vessel is floating in drydocked
When a vessel is in dry dock, the areas where transverse stresses are typically highest include:
- Flat of Bottom:
- Loss of Buoyant Support: The flat of the bottom, especially between the keel blocks, experiences significant stress due to the concentrated load of the vessel’s weight and the lack of buoyant support in that area.
- Sagging: This can lead to sagging or hogging of the hull, putting the bottom plating and associated structures under considerable stress.
- Turn of the Bilge:
- Stress Concentration: The turn of the bilge, where the flat of the bottom transitions to the ship’s side, is an area of stress concentration due to the change in hull shape and the load distribution.
- Potential for Deformation: This area can be prone to deformation or cracking if not adequately reinforced.
- Connections of Transverse Bulkheads:
- Load Transfer: Transverse bulkheads transfer loads from the deck and sides to the bottom structure. The connections between the bulkheads and the hull plating, particularly at the turn of the bilge, can experience high stresses.
- Areas with Concentrated Loads:
- Machinery and Equipment: Areas with heavy machinery or equipment, such as the engine room or cargo holds, can experience localized stress concentrations due to the weight acting on the hull structure.
- Areas with Longitudinal Stress Concentrations:
- Deck Openings: Large deck openings, such as hatchways or cargo holds, can create stress concentrations in the deck structure, which can also induce transverse stresses in the supporting beams and frames.
Why these areas are critical:
- Structural Failure: If these areas are not designed and constructed with sufficient strength, they can be susceptible to cracking, buckling, or even structural failure during dry-docking.
- Fatigue: The repeated stress cycles experienced during dry-docking can contribute to fatigue cracking over time, especially in areas of stress concentration.
Mitigating Measures:
- Adequate Support: The dry dock should have a sufficient number of keel blocks and side blocks to distribute the ship’s weight evenly and prevent excessive sagging.
- Proper Block Placement: The blocks should be carefully positioned based on the ship’s docking plan to minimize stress concentrations and provide adequate support for the hull structure.
- Strong Transverse Framing: A robust transverse framing system, including frames, beams, and bulkheads, helps to resist the docking stresses and maintain the hull’s shape.
- Reinforcements: Adding extra stiffeners, brackets, or doublers in critical areas can further strengthen the structure and reduce stress concentrations.
- Monitoring and Adjustments: Continuous monitoring of the ship’s position and stress levels during dry-docking allows for timely adjustments to prevent damage.
In conclusion, understanding the areas of maximum transverse stress in a dry-docked vessel is essential for ensuring its structural integrity and safety during this critical maintenance operation. By addressing these stress concentrations through proper design, construction, and dry-docking procedures, shipbuilders and operators can minimize the risk of damage and ensure the continued seaworthiness of the vessel.
Structure that resists the stress
The structure that resists transverse stresses in a vessel’s hull is primarily the transverse framing system, working in conjunction with the hull plating and bulkheads. This system is designed to provide strength and rigidity across the ship’s breadth, preventing excessive deformation or failure under various loading conditions.
Here’s a breakdown of the key components and how they contribute to resisting transverse stresses:
- Frames:
- Arrangement: These are vertical or slightly curved members running across the ship’s width, spaced at regular intervals along its length.
- Function: They act as ribs, providing support for the hull plating and resisting the inward pressure exerted by the water.
- Types:
- Web Frames: Solid plate frames, typically used in areas of high stress or where openings in the hull are required.
- Angle Frames: L-shaped frames, commonly used in less stressed areas.
- Bulb Frames: T-shaped frames with a bulbous section at the bottom, offering greater strength and stiffness.
- Beams:
- Arrangement: These are horizontal members running across the ship’s width, connecting the frames and supporting the decks.
- Function: They resist the downward forces from cargo, machinery, and other loads, as well as the upward forces from buoyancy. They also help to distribute the loads more evenly to the frames and hull plating.
- Types:
- Transverse Beams: Run across the ship’s breadth.
- Longitudinal Beams: Run along the ship’s length, providing additional support and stiffness.
- Bulkheads:
- Arrangement: Vertical partitions that divide the ship into separate compartments.
- Function: While primarily designed for watertight subdivision or fire protection, bulkheads also contribute to transverse strength by acting as vertical webs or girders, preventing the hull from racking or twisting.
- Hull Plating:
- Arrangement: The outer skin of the ship, consisting of steel plates welded together.
- Function: While primarily providing watertightness, the hull plating also acts as a stressed skin, contributing to the overall strength and rigidity of the hull, including its resistance to transverse stresses.
How these components work together:
- Integrated System: The frames, beams, bulkheads, and hull plating work together as an integrated system to resist transverse stresses.
- Load Distribution: The loads acting on the hull are transferred through the plating to the frames and beams, which then distribute the loads to the bulkheads and ultimately to the keel and bottom structure.
- Stiffness and Rigidity: The interconnected network of frames, beams, and bulkheads provides transverse stiffness and prevents the hull from deforming excessively under load.
Additional Factors:
- Material Strength: The strength of the steel used for the hull plating and framing members is crucial for resisting stresses.
- Welding Quality: Proper welding techniques and quality control are essential to ensure strong and reliable connections between the structural components.
- Corrosion Prevention: Corrosion can weaken the hull structure, so effective corrosion prevention measures, such as coatings and cathodic protection, are important for maintaining its strength.
In conclusion, the transverse framing system, comprising frames, beams, bulkheads, and hull plating, is the primary structure that resists transverse stresses in a vessel’s hull. This integrated system ensures the vessel’s strength, stability, and ability to withstand the various loads and forces it encounters during its operational life.