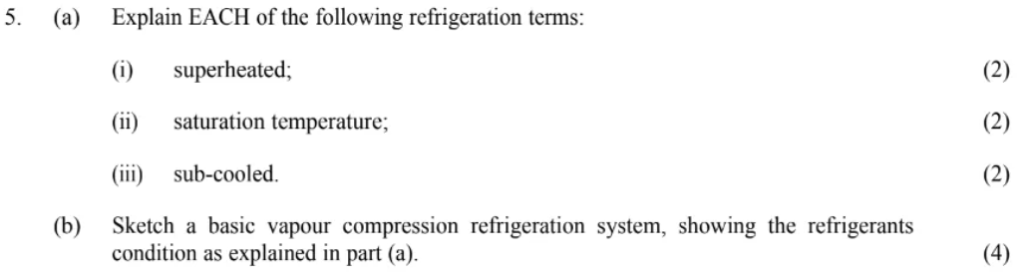
- (a) Explain EACH of the following refrigeration terms:
(i) superheated;
(ii) saturation temperature;
(iii) sub-cooled.
(b) Sketch a basic vapour compression refrigeration system, showing the refrigerants condition as explained in part (a).
Superheated
In refrigeration, superheat refers to the difference between the actual temperature of a refrigerant vapor and its saturation temperature at the same pressure.
Understanding the Concept
- Saturation Temperature: This is the temperature at which a refrigerant changes its phase from liquid to vapor (or vice-versa) at a given pressure. It’s a crucial property in refrigeration cycles, as the refrigerant absorbs heat during evaporation (liquid to vapor) and releases heat during condensation (vapor to liquid).
- Superheated Vapor: When the refrigerant vapor’s temperature is higher than its saturation temperature, it’s said to be superheated. This additional heat beyond the phase change point is the superheat.
Why is Superheat Important in Refrigeration?
- Compressor Protection: Compressors are designed to compress vapor, not liquid. If liquid refrigerant enters the compressor, it can cause severe damage due to its incompressibility. Superheat ensures that all the liquid refrigerant has evaporated in the evaporator before reaching the compressor, thus safeguarding it.
- System Efficiency: Superheat ensures that only vapor enters the compressor, maximizing the compressor’s efficiency and the system’s cooling capacity.
- Troubleshooting: Measuring superheat helps diagnose potential problems within the refrigeration system:
- High Superheat: May indicate insufficient refrigerant charge, a restriction in the system, or a faulty expansion valve.
- Low Superheat: Can point to an overcharge of refrigerant or a malfunctioning thermostatic expansion valve, potentially leading to liquid floodback to the compressor.
How Superheat is Measured
- Measure Pressure and Temperature: At the outlet of the evaporator, measure both the refrigerant’s pressure and temperature.
- Determine Saturation Temperature: Use a pressure-temperature chart (specific to the refrigerant) to find the saturation temperature corresponding to the measured pressure.
- Calculate Superheat: Subtract the saturation temperature from the measured actual temperature. The result is the superheat value, typically expressed in degrees Fahrenheit or Celsius.
Typical Superheat Range
The desired superheat range varies depending on the specific system and refrigerant used, but generally, it’s maintained between 10 to 20 degrees Fahrenheit (5 to 11 degrees Celsius) at the evaporator outlet.
In conclusion, superheat is a vital concept in refrigeration. It ensures the safe and efficient operation of the system by protecting the compressor and maximizing cooling capacity. By understanding and managing superheat, technicians can maintain the optimal performance of refrigeration systems and avoid costly breakdowns.
Saturation
In refrigeration, the saturation temperature is the temperature at which a refrigerant changes its phase from liquid to vapor (evaporation) or from vapor to liquid (condensation) at a given pressure. It’s a crucial concept because the entire refrigeration cycle revolves around these phase changes, which allow the refrigerant to absorb and release heat, thereby cooling the desired space.
Key Points:
- Pressure-Temperature Relationship: For every refrigerant, there’s a direct relationship between its pressure and saturation temperature. This relationship is depicted in a Pressure-Temperature (P-T) chart or diagram, unique to each refrigerant.
- Evaporation: In the evaporator, the refrigerant absorbs heat from the surroundings and changes from liquid to vapor at its saturation temperature corresponding to the evaporator pressure.
- Condensation: In the condenser, the refrigerant releases heat to the surroundings and changes from vapor to liquid at its saturation temperature corresponding to the condenser pressure.
- Superheat and Subcooling:
- Superheat is the temperature difference between the actual refrigerant vapor temperature and its saturation temperature at the same pressure. It ensures that only vapor enters the compressor, preventing damage.
- Subcooling is the temperature difference between the saturation temperature and the actual liquid refrigerant temperature at the same pressure. It ensures that only liquid enters the expansion valve, improving system efficiency.
Example:
- For R-134a refrigerant, if the pressure in the evaporator is 30 psig, its saturation temperature (from the P-T chart) is approximately 35°F.
- If the actual refrigerant temperature at the evaporator outlet is measured to be 45°F, then the superheat is 10°F.
- Similarly, if the pressure in the condenser is 120 psig, the saturation temperature is around 100°F. If the liquid refrigerant leaving the condenser is at 90°F, the subcooling is 10°F.
Significance:
- System Design and Operation: Understanding saturation temperature is essential for designing and operating refrigeration systems. Engineers and technicians use P-T charts to select appropriate components, set operating pressures, and troubleshoot system problems.
- Charging and Performance: Measuring and controlling superheat and subcooling, which are directly related to saturation temperature, are crucial for ensuring the system is properly charged with refrigerant and operating efficiently.
In essence, the saturation temperature is a fundamental property in refrigeration, defining the phase change points of the refrigerant and guiding the design, operation, and maintenance of refrigeration systems.
Sub-cooled
In refrigeration, subcooling refers to the condition where a refrigerant exists in its liquid phase at a temperature below its saturation temperature for a given pressure.
Let’s break this down further:
- Saturation Temperature: This is the temperature at which a refrigerant changes its phase from liquid to vapor (or vice versa) at a given pressure. It’s a crucial point in the refrigeration cycle.
- Subcooled Liquid: When the liquid refrigerant’s temperature is lower than its saturation temperature at that same pressure, it’s said to be subcooled. The difference between the saturation temperature and the actual liquid temperature is the degree of subcooling.
Why Subcooling Matters:
- Improved Efficiency:
- Subcooling ensures that only liquid refrigerant enters the expansion valve. This prevents any premature flashing (vaporization) of the refrigerant, which would reduce the cooling capacity in the evaporator.
- In essence, subcooling allows the refrigerant to absorb more heat in the evaporator, improving the system’s overall efficiency.
- System Stability:
- Subcooling helps prevent vapor bubbles from forming in the liquid line, which can cause erratic operation of the expansion valve and reduce system performance.
How Subcooling Occurs:
- Condenser: The primary location for subcooling is in the condenser, after the refrigerant has condensed from vapor to liquid.
- Additional Subcooler: In some systems, a separate subcooler might be used after the condenser to further decrease the refrigerant’s temperature.
Measuring Subcooling:
- Measure the pressure at the outlet of the condenser (or subcooler).
- Use a pressure-temperature chart (specific to the refrigerant) to find the corresponding saturation temperature.
- Measure the actual temperature of the liquid refrigerant at the same point.
- Subcooling = Saturation Temperature – Actual Liquid Temperature
Typical Subcooling Range:
- A typical subcooling range is between 10 to 20 degrees Fahrenheit (5 to 11 degrees Celsius). However, the ideal value can vary depending on the system design and refrigerant used.
In summary, subcooling is a desirable condition in refrigeration systems as it ensures efficient operation and system stability. It allows the refrigerant to maximize its heat absorption capacity in the evaporator, leading to better cooling performance.
Basic vapour compression refrigeration system
Key Components and Refrigerant’s State:
- Compressor:
- Refrigerant enters as a low-pressure, low-temperature superheated vapor.
- The compressor compresses this vapor, increasing its pressure and temperature.
- Refrigerant leaves as a high-pressure, high-temperature superheated vapor.
- Condenser:
- Refrigerant enters as a high-pressure, high-temperature superheated vapor.
- Heat is rejected to the surroundings (air or water), causing the refrigerant to condense.
- Refrigerant leaves as a high-pressure, warm liquid (ideally with some subcooling).
- Expansion Valve (or Metering Device):
- Refrigerant enters as a high-pressure liquid.
- The valve creates a restriction, causing a significant pressure drop.
- This pressure drop forces some of the refrigerant to evaporate, cooling the remaining liquid.
- Refrigerant leaves as a low-pressure, low-temperature mixture of liquid and vapor.
- Evaporator:
- Refrigerant enters as a low-pressure, low-temperature mixture of liquid and vapor.
- It absorbs heat from the space being cooled, causing the remaining liquid to evaporate completely.
- Refrigerant leaves as a low-pressure, low-temperature superheated vapor, ready to enter the compressor again, completing the cycle.
Visual Representation:
Compressor
(Vapor -> High-Pressure Vapor)
|
|
Condenser
(Vapor -> Liquid)
|
|
Expansion Valve
(Liquid -> Liquid/Vapor Mixture)
|
|
Evaporator
(Liquid/Vapor -> Vapor)
|
|
(Back to Compressor)
Key Points:
- Phase Changes: The refrigerant undergoes phase changes (liquid to vapor and vice-versa) to facilitate heat transfer.
- Pressure and Temperature: The refrigerant’s pressure and temperature change throughout the cycle, following the pressure-temperature relationship specific to the refrigerant used.
- Superheat and Subcooling:
- Superheat at the evaporator outlet ensures only vapor enters the compressor.
- Subcooling at the condenser outlet ensures only liquid enters the expansion valve, improving efficiency.
- Heat Transfer:
- Heat is absorbed from the cooled space in the evaporator.
- Heat is rejected to the surroundings in the condenser.
Remember: This is a simplified description of a basic vapor compression cycle. Real-world systems can be more complex, incorporating additional components like receivers, accumulators, and various control devices for optimal performance and safety.