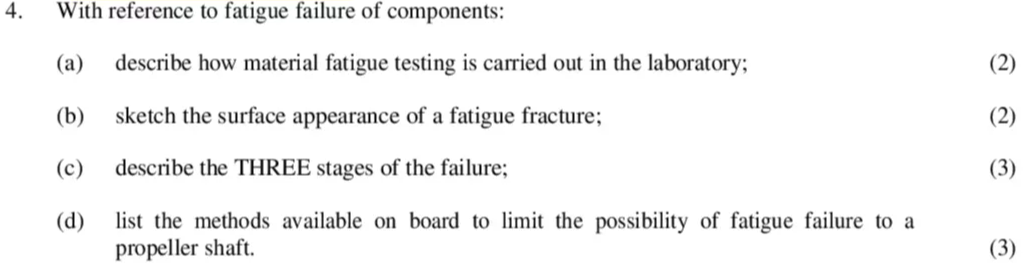
(a) Material Fatigue Testing in Lab:
Laboratory fatigue testing simulates real-world cyclic loading to assess a material’s resistance to fatigue failure. Common methods include:
- Rotating Beam Test: A rotating cantilever beam experiences cyclic bending stresses as it spins. The number of cycles to failure at a specific stress level determines the fatigue strength.
- Axial Fatigue Test: A specimen is subjected to cyclic tension and compression loading to evaluate fatigue performance under these conditions.
- Push-Pull Test: A specimen is pulled in one direction and then pushed back in the opposite direction, mimicking cyclic axial stresses.
- Four-Point Bending Test: A specimen is supported at two points and loaded at two other points, creating a constant bending moment region to study fatigue behaviour.
These tests measure fatigue life (number of cycles to failure) at various stress levels, generating S-N curves that map stress vs. number of cycles to failure. This data allows material selection, design optimization, and prediction of fatigue life in real applications.
(b) Sketch of Fatigue Fracture Surface:
A fatigue fracture exhibits distinct features:
- Initiation Site: Often a surface defect like a scratch, corrosion pit, or inclusion acts as the starting point for micro-cracks.
- Beach Marks: Concentric semicircular ridges around the initiation site represent progressive crack growth under each loading cycle.
- Final Fracture Zone: This area displays rougher fracture features due to rapid crack propagation leading to final failure.
Sketch: Imagine a circular area with concentric semi-circular ridges around a central point. The ridges become larger and closer as they move away from the centre. The edge shows a rougher fractured area.
(c) Three Stages of Fatigue Failure:
- Crack Initiation: Micro-cracks form and propagate at microscopic levels from stress concentrations at defects or inhomogeneities within the material.
- Crack Propagation: The micro-cracks gradually grow under repeated loading, creating beach marks on the fracture surface. The rate of crack growth depends on stress level, material properties, and environmental factors.
- Final Fracture: When the crack reaches a critical size, it can no longer withstand the applied stress and rapidly propagates across the remaining cross-section, leading to final failure.
(d) Mitigation Methods for Propeller Shaft Fatigue:
Onboard strategies to limit propeller shaft fatigue failure include:
- Preventative Maintenance: Regularly inspecting the shaft for surface defects, cracks, and corrosion allows for early detection and repairs, preventing further crack growth.
- Vibration Control: Minimising vibrations through shaft balancing, alignment adjustments, and dampening devices reduces dynamic loading and stress concentrations.
- Propeller Maintenance: Ensuring proper propeller balance and blade pitch minimises uneven loading on the shaft.
- Material Selection: Using fatigue-resistant materials can improve the shaft’s overall fatigue life.
- Operational Monitoring: Monitoring stress levels, vibrations, and shaft RPM during operation allows for adjustments to operating conditions and early detection of potential fatigue concerns.
By implementing these mitigation strategies, the risk of fatigue failure in a propeller shaft can be significantly reduced, ensuring its continued reliable operation and preventing catastrophic consequences.